Qubits
In conventional computing, information is encoded as binary digits or ‘bits’ – a basic unit of information – that can be represented as either a ‘0’ or ‘1’. In quantum computing the equivalent unit is a quantum bit or ‘qubit’, which can exist either in a state uniquely as ‘0’ or ‘1’ or as a simultaneous combination of both ‘0’ and ‘1’, owing to superposition. A register can be constructed from multiple qubits, which can then become correlated with each other in a subtle and powerful way that cannot exist in the ordinary, classical world — this is called quantum entanglement. The combination of superposition and entanglement means that a quantum register can encode information in a large number of states simultaneously. This feature is crucial in enabling a quantum computer’s power, allowing it to perform computations for tasks that are intractable for even the largest current supercomputers.
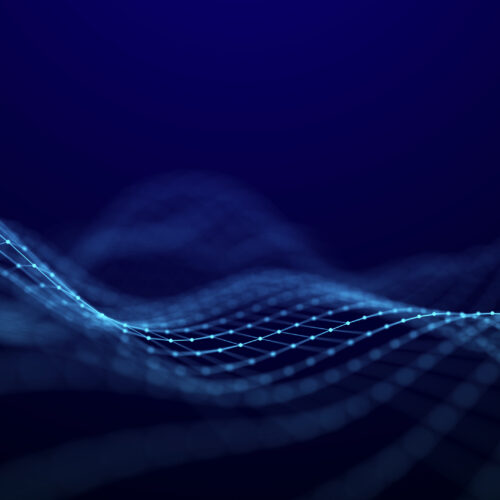
Full stack & architecture
Quantum computers can have different architectures, being based on alternative designs and constructed in different ways. As with conventional computers, there are several hardware and software layers involved in constructing a quantum computer, that go from the processor through to the user interface – this is referred to as the full stack.
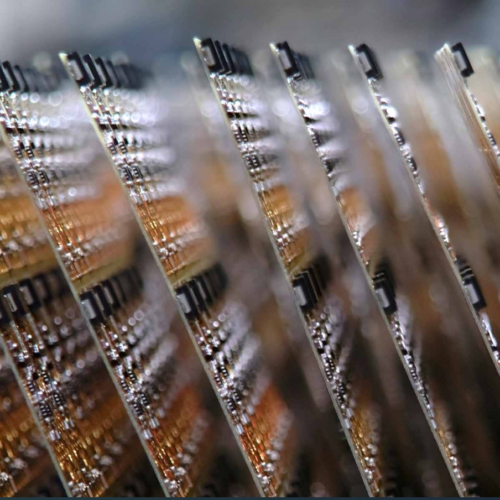
Applications
The availability of quantum computing is expected to impact many sectors, enabling improvements in efficiency, productivity and competitiveness, as well as the creation of new products and services. Promising early applications include: the modelling and analysis of more complex molecules to accelerate the discovery of new materials and pharmaceuticals, the optimisation of complex planning and scheduling for logistics enhancing the design and manufacturing processes in sectors such as aerospace and automotive the optimisation of networks: the routing of traffic, telecommunications and energy distribution.
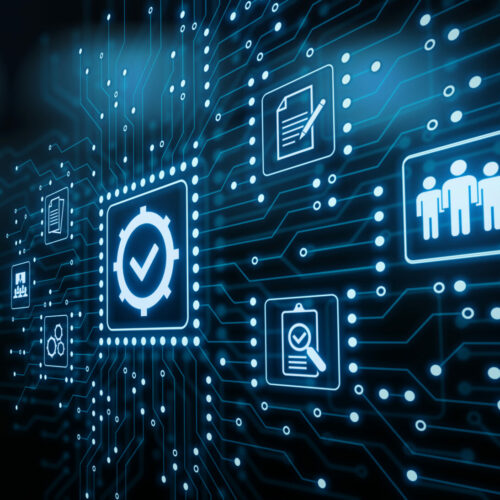
Technical challenges
There are several candidate hardware architectures on which to base quantum computers, each with their own merits and at various stages of technology maturity. The common feature is that physical qubits are inherently fragile, requiring precise control and protection from the external environment. The states need to be as long-lived as possible and the operations on them high-fidelity, otherwise errors accumulate, and more computational resource is required for error correction. Architectures that require limited or even no error correction are being investigated. Technical hurdles have continually been overcome as the field has advanced, but there are significant engineering challenges that lie ahead in scaling to ever greater numbers of qubits with low noise. We also need to learn more about the merits of the different hardware architectures as quantum computers are scaled. A real breakthrough would be to build and demonstrate quantum computers that provide enhanced performance for a range of different tasks. In the near term, we can explore how to tackle some of these challenges by developing NISQ (noisy intermediate-scale quantum) machines, which can provide a pathway to a general-purpose quantum computer in the future.
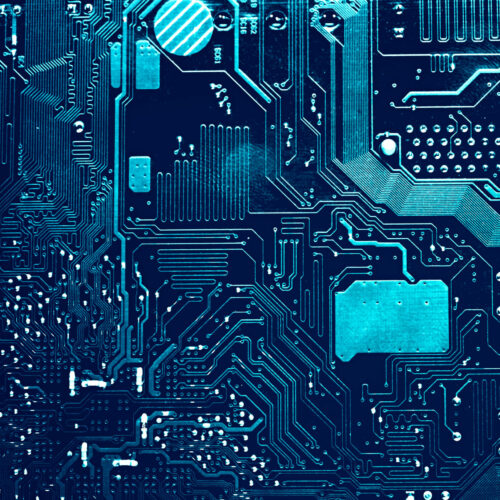